Quantum Computing Quantum Image Processing: The Future of Image Analysis
- Rushi Joshi
- Jan 28, 2024
- 5 min read
I want to thank my friend Britant for his help in drafting this article.
He's a Researcher working on Quantum Computing and Algorithms. - Linkedin

1. Introduction
Quantum computing[QuC] is at the forefront of modern technology, promising to revolutionize industries by solving complex problems far beyond the capabilities of classical computers. This field leverages the principles of quantum mechanics, particularly the phenomena of Superposition - {Quantum particles exist in multiple states at a single instance of time, which vastly increases the amount of information that can be stored in a single bit, unlike just 0/1} and Entanglement - {Quantum particles are connected in a fuzzy manner which makes information related by default minimising the analytical efforts}, allowing quantum computers to process vast amounts of data simultaneously.
Quantum computers are not just theoretical marvels but are gradually becoming a part of the scientific toolkit for exploring fundamental questions in physics. For instance, IBM's advancements allow for longer qubit coherence times, enabling more complex quantum states and operations and opening new avenues for scientific inquiry, such as simulating intricate quantum phenomena of solving higher dimensional problems in Quantum many-body systems or classical NP-Hard Problems such as Portfolio Optimisation.
2. Role of QuCs in Image Processing
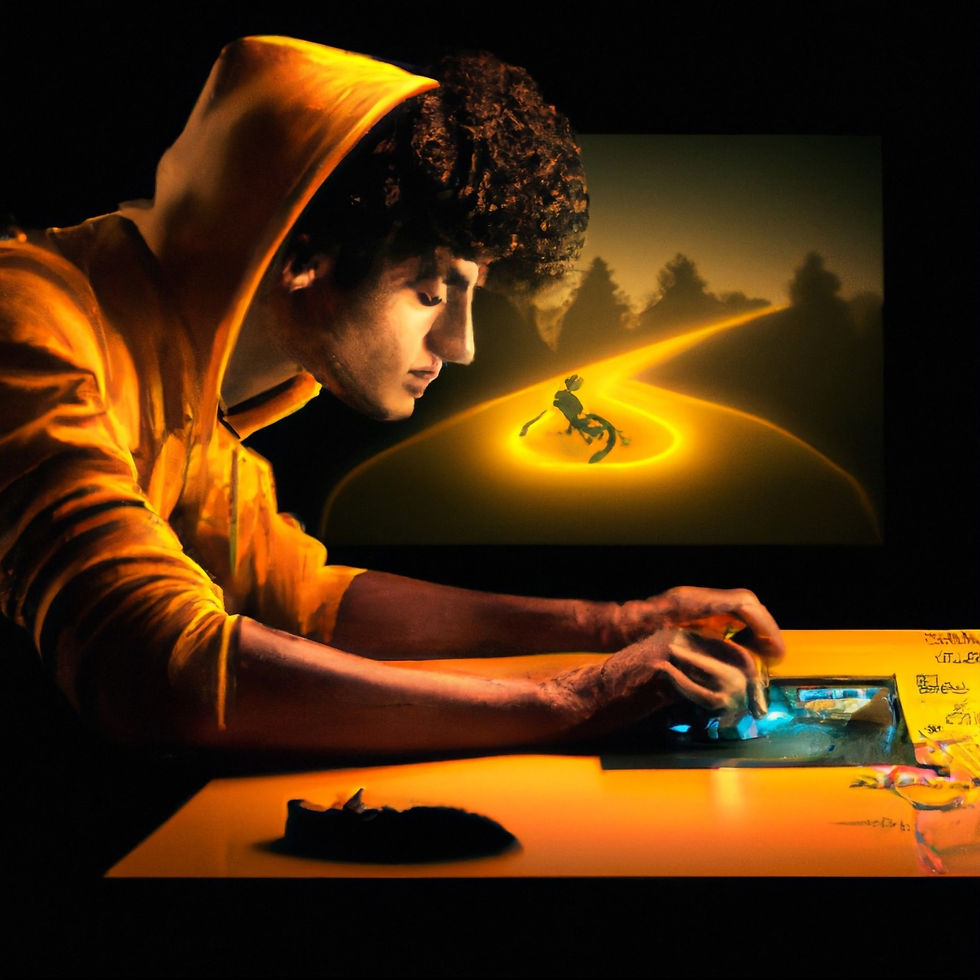
Image processing, a branch of digital signal manipulation, employs sophisticated algorithms to enhance, analyze, and interpret images. It's pivotal in sectors like healthcare, where it enhances medical imaging and autonomous vehicle technology, which aids navigation and obstacle detection. Techniques include image enhancement, which improves visual clarity, and image restoration, which corrects imperfections. Image compression reduces file size for efficient storage and transmission, while image encryption secures data against unauthorized access. Furthermore, image segmentation divides images into meaningful structures, which is essential in object recognition tasks. In object classification, algorithms categorize detected objects, supporting automated systems in decision-making. Overall, image processing converts raw visual data into actionable insights, driving advancements across diverse fields by enabling machines to effectively understand and interact with the visual world.
IMAGE COMPRESSION
Image compression is a pivotal process in digital image processing, aimed at reducing the spatial data representation of images and optimizing storage efficiency and transmission speed. It bifurcates into two distinct methodologies:
1. Lossless Compression: This technique ensures data integrity, allowing the exact original image to be reconstructed from the compressed data.
2. Lossy Compression: Prioritizing compression ratio, lossy methods yield significantly reduced file sizes by selectively discarding less critical data, a trade-off that induces irreversible quality degradation.
IMAGE ENCRYPTION
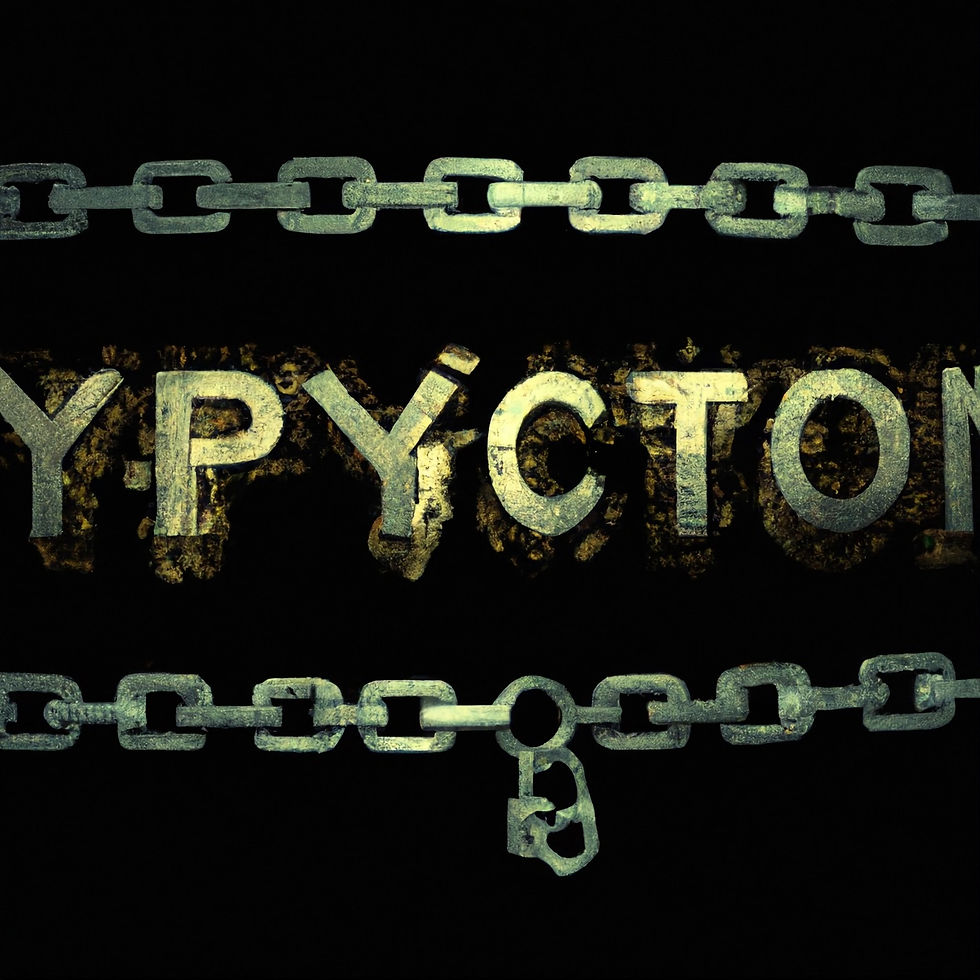
Image encryption is a method of safeguarding digital photos to maintain their privacy and stop unwanted access. It uses cryptographic techniques to change an image's information such that only authorized persons possessing the necessary decryption keys may restore it to its original state.
Quantum computers store images in qubits, in contrast to classical image processing. Effectively using both the superposition state and the entangled state of quantum computing, quantum computers can handle large amounts of data in parallel. On a classical computer, the complexity of storing n-bit long sequences is O(n × 2^n), whereas on a quantum computer, it is just O(n). Quantum computers work on sequences in a way that classical computers cannot; it requires many repeated arithmetic circuits or sequential calculations with a complexity of O(2^n). One example of an operation that quantum computers can perform on each bit simultaneously. The fact that only O(1) is required helps speed up techniques for image processing that include several simultaneous computations. The algorithm's efficiency is considerably increased since less space is needed to store the image, and less circuit scale is needed to process the image. About three components are needed for quantum image processing. A digital image is represented on a quantum computer using a quantum algorithm. Then, the image is again converted into a classical image. Images must be stored in a quantum system for a quantum computer to process them. Numerous quantum image representation techniques have been developed to address such issues. DCT-EFRQI (Direct Cosine Transform Efficient Flexible Representation of Quantum Image) is one of the most recently developed techniques that can handle image compression, having a large number of pixels. This approach can significantly save
computational time and the number of qubits required for image compression.
a. Quantum Image Encryption-
Several encryption techniques exist, and the common thing in all techniques is that encryption occurs via quantum mechanical operations. One of the simplest ways to encrypt an image is via rotation gates; the qubit on the Bloch sphere is rotated at any angle using the quantum rotation gate, which is useful for image encryption.
In 2018, Liu et al. presented a new colour image encryption and decryption technique that relies on quantum Fourier transform (QFT) and random qubit rotation. Qubit rotation uses the quantum Fourier transform to function once in the spatial and frequency domains.
b. Quantum Image Compression - While representing an image, quantum compression offers better reproduction because we use qubits to store the image’s pixel information. This replaces the classical way of storing pixels in an array. As the no. of qubits grows in ‘n’, we get 2^n no. of states to store the information. This offers an exponential advantage over the classical array style of storing images. Moreover, the number of processing images becomes bulky, leading to the algorithm’s complexity in classical computation. In classical computation, processing those huge images requires more memory and hardware. Quantum image processing delivers a quadratic speed up when contrasted with classical computation.
3. Current Status of Technology and Market
The quantum computing market is witnessing substantial growth and is poised to transform numerous industries. The global market size for quantum computing was valued at USD 717.3 million in 2022 and is projected to grow to USD 6,528.8 million by 2030, exhibiting a CAGR of 32.1% during the forecast period. Different sources provide varying figures for market size and growth rates, reflecting the industry's dynamic and rapidly evolving nature. For instance, one estimate puts the market at USD 866 million in 2023 with expectations to reach USD 4,375 million by 2028, growing at a CAGR of 38.3% from 2023 to 2028, while another suggests the market could reach USD 143.44 billion by 2032.
Factors Influencing the Market:
Technological Advancements: Breakthroughs in quantum technology, such as IBM's 433-qubit quantum processor and plans for a 4,000-qubit processor by 2025, are significant market growth drivers.
Investments and Funding: The sector has seen record investments, with quantum technology start-ups receiving their highest funding levels in 2022, totalling $2.35 billion.
Public Sector Support: Governments worldwide are investing heavily in quantum technology. For example, in 2022, the U.S. committed an additional $1.8 billion, the European Union $1.2 billion, and Canada $100 million.
Educational Initiatives: Universities are increasingly offering master’s degree programs in quantum technologies, helping to narrow the talent gap and fuel industry growth.
Job Opportunities and Roles: The quantum computing sector offers a variety of roles, many of which require specialized knowledge and advanced degrees. Key positions include:
Qubit Researchers: Specialists in qubit modalities who focus on the design, integration, and layout of quantum systems. These roles typically require a Ph.D. in Physics, Computer Science, or Engineering.
Quantum Control Researchers: Experts in controlling quantum gates and interactions within quantum systems, also requiring a PhD in Physics with experience in quantum control and information.
Quantum Error Correction Researchers: Professionals who develop codes and protocols for reliable quantum information storage and processing, usually requiring a Ph.D. in Physics, specifically in Quantum Technologies or Quantum Information Theory.
Quantum Algorithms Researchers: Individuals focusing on computational problems solvable through quantum phenomena. This role requires a Ph.D. in Mathematics, Theoretical Physics, or Computer Science.
Quantum Engineers: Focused on developing quantum computer architectures and quantum sensors, with starting salaries ranging between $90,000 to $100,000.
The quantum computing field offers promising career opportunities, with roles like Quantum Cryptography Scientists and Quantum Computing Software Engineers commanding salaries starting at $100,000 and ranging between $105,000 and $125,000, respectively.
NOTE:- The data here has been taken from trusted journals and websites.
.
Comments